Muscle Contraction: Molecular and Cellular Physiology
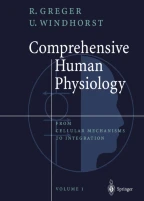
Skeletal muscles are by far the largest organ of the human body, accounting for up to 40%–50% of the body weight. In the past much of our knowledge on muscle contraction and relaxation was obtained from experiments with isolated frog muscle or single muscle fibers stimulated with electric pulses. If a vertically suspended muscle is fixed at the upper end and the lower end is loaded with a weight, the muscle fibers become stretched; however, if they are stimulated with a single electric pulse, the muscle fibers respond with an isotonic twitch, thereby lifting the load and performing mechanical work. If, on the other hand, both ends of the muscle are firmly held, the stimulated muscle fibers cannot shorten, but instead generate force in an isometric contraction. The duration of the twitch may vary, depending on whether fast- or slow-twitch muscle fibers have been used for the experiment. If the stimulation is repeated at short intervals of, say, 50 ms, twitches superimpose and even fuse to a smooth tetanus, developing a force of up to 20–30 N per cm 2 fiber cross-section.
This is a preview of subscription content, log in via an institution to check access.
Access this chapter
Subscribe and save
Springer+ Basic
€32.70 /Month
- Get 10 units per month
- Download Article/Chapter or eBook
- 1 Unit = 1 Article or 1 Chapter
- Cancel anytime
Buy Now
Price includes VAT (France)
eBook EUR 319.93 Price includes VAT (France)
Softcover Book EUR 400.89 Price includes VAT (France)
Tax calculation will be finalised at checkout
Purchases are for personal use only
Preview
Similar content being viewed by others
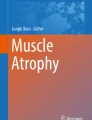
Myofibers
Chapter © 2018
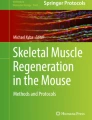
Assessment of the Contractile Properties of Permeabilized Skeletal Muscle Fibers
Chapter © 2016
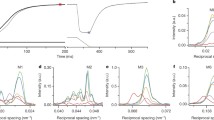
Force generation by skeletal muscle is controlled by mechanosensing in myosin filaments
Article 11 November 2015
References
- Allen DG, Blinks JR (1979) The interpretation of light signals from aequorin-injected skeletal and cardiac muscle cells: a new method of calibration. In: Ashley CC, Campbell AK (eds) Detection and measurement of free Ca 2+ in cells. Elsevier/North-Holland Biomedical, Amsterdam, pp 159–174 Google Scholar
- Allen DG, Westerblad H, Lee JA, Lännergren J (1992) Role of excitation contraction coupling in muscle fatigue. Sports Med 13:116–126 PubMedCASGoogle Scholar
- Almers W, Palade PT (1981) Slow calcium and potassium currents across frog muscle membrane: measurements with a vaseline-gap technique. J Physiol (Lond) 312:159–176 PubMedCASGoogle Scholar
- Ashley CC, Mulligan IP, Trevor JL (1991) Ca 2+ and activation mechanisms in skeletal muscle. Q Rev Biophys 24:1–73 PubMedCASGoogle Scholar
- Bagni MA, Cecchi G, Schoenberg M (1988) A model of force production that explains the lag between crossbridge attachment and force after electrical stimulation of striated muscle fibres. Biophys J 54:1105–1114 PubMedCASGoogle Scholar
- Bagshaw CR (1992) Muscle contraction, 2nd edn. Chapman and Hall, London Google Scholar
- Bailey K (1942) Myosin and adenosinetriphosphatase. Biochem J 36:121–139 PubMedCASGoogle Scholar
- Blinks JR, Rüdel R, Taylor SR (1978) Calcium transients in isolated amphibian skeletal muscle fibres: detection with aequorin. J Physiol 277:291–323 PubMedCASGoogle Scholar
- Block BA, Imagawa T, Campbell KP, Franzini-Armstrong C (1988) Structural evidence for direct interaction between the molecular components of the transverse tubule/SR junction in skeletal muscle. J Cell Biol 107:2587–2600 PubMedCASGoogle Scholar
- Brenner B (1988) Effect of Ca 2+ on crossbridge turnover kinetics in skinned single rabbit psoas fibres: implications for regulation of muscle contraction. Proc Natl Acad Sci U S A 85:3265–3269 PubMedCASGoogle Scholar
- Brenner B (1990) Muscle mechanics and biochemical kinetics. In: Squire JM (ed) Molecular mechanisms in muscular contraction. Macmillan, New York Google Scholar
- Brenner B, Eisenberg E (1986) Rate of force generation in muscle: correlation with actomyosin ATPase in solution. Proc Natl Acad Sci U S A 83:3542–3546 PubMedCASGoogle Scholar
- Brenner B, Yu LC (1993) Structural changes in the actomyosin cross-bridges associated wit force generation. Proc Natl Acad Sci U S A 90:5252–5256 PubMedCASGoogle Scholar
- Buller AJ, Eccles JC, Eccles RM (1960) Interactions between motoneurones and muscles in respect of the characteristic speeds of their responses. J Physiol (Lond) 150:417–439 PubMedCASGoogle Scholar
- Cuda G, Fananapazir L, Zhu WS, Sellers J, Epstein ND (1993) Skeletal muscle expression and abnormal function of β-myosin in hypertrophic cardiomyopathy. J Clin Invest 91:2861–2865 PubMedCASGoogle Scholar
- Davies RE (1964) Adenosine triphosphate breakdown during single muscle contractions. Proc R Soc Lond [Biol] 160:480–485 CASGoogle Scholar
- Ebashi S, Endo M (1968) Calcium ion and muscle contraction. Prog Biophys Mol Biol 18:125–183 Google Scholar
- Eisenberg E, Hill TL (1985) Muscle contraction and free energy transduction in biological systems. Science 227:999–1006 PubMedCASGoogle Scholar
- Eisenberg E, Moos C (1970) Actin activation of heavy meromyosin adenosine triphosphatase. Dependence on adenosine triphosphatase and actin concentrations. J Biol Chem 245:2451–2456 PubMedCASGoogle Scholar
- Feldmeyer D, Melzer W, Pohl B, Zöllner P (1990) Fast gating kinetics of the slow Ca 2+ current in cut skeletal muscle fibres of the frog. J Physiol (Lond) 425:347–367 PubMedCASGoogle Scholar
- Filo RS, Bohr DF, Rüegg JC (1965) Glycerinated skeletal and smooth muscle. Calcium and magnesium dependence. Science 147:1581–1583 PubMedCASGoogle Scholar
- Finer JT, Simmons RM, Spudich JA (1994) Single myosin molecule mechanics: piconewton forces and nanometre steps. Nature 368:113–119 PubMedCASGoogle Scholar
- Ford LE, Huxley AF, Simmons RM (1986) Tension transients during the rise of tetanic tension in frog muscle fibres. J Physiol (Lond) 372:595–609 PubMedCASGoogle Scholar
- Franzini-Armstrong C, Peachey LD (1981) Striated muscle-contractile and control mechanisms. J Cell Biol 91:166s-186s PubMedGoogle Scholar
- Gergely J, Grabarek Z, Leavis PC, Strasburg G, Tao T, Wang CLA (1988) Transmission of the Ca 2+ -regulatory signal in skeletal muscle thin filaments. Adv Exp Med Biol 226:155–163 PubMedCASGoogle Scholar
- Gillis JM (1985) Relaxation of vertebrate skeletal muscle. A synthesis of the biochemical and physiological approaches. Biochim Biophys Acta 811:97–145 PubMedCASGoogle Scholar
- Goldman YE, Hibberd MG, McCray JA, Trentham DR (1982) Relaxation of muscle fibres by photolysis of caged ATP. Nature 300:701–705 PubMedCASGoogle Scholar
- González-Serratos H (1971) Inward spread of activation in vertebrate muscle fibres. J Physiol (Lond) 212:777–799 PubMedGoogle Scholar
- Gordon AM, Yates LD (1992) Regulatory mechanism of contraction in skeletal muscle. In: Sugi H (ed) Muscle contraction and cell motility. Molecular and cellular aspects. Springer Berlin Heidelberg New York (Advances in comparative and environmental physiology, vol 12) Google Scholar
- Güth K, Potter JD (1987) Effect of rigor and cycling crossbridges on the structure of troponic C and on the calcium affinity of the calcium specific regulatory sites in skinned rabbit psoas fibres. J Biol Chem 262:13627–13635 PubMedGoogle Scholar
- Hasselbach W, Makinose M (1961) Die Calciumpumpe der “Erschlaffungsgrana” des Muskels und ihre Abhägigkeit von der ATP-Spaltung. Biochem Z 333:518–528 PubMedCASGoogle Scholar
- Hasselbach W, Makinose M (1962) ATP and active transport. Biochem Biophys Res Commun 7:132–136 PubMedCASGoogle Scholar
- Heilbrunn LV, Wiercinski FJ (1947) The action of various cations on muscle protoplasm. J Cell Comp Physiol 29: 15–32 CASGoogle Scholar
- Hellam DC, Podolsky RJ (1969) Force measurements in skinned muscle fibres. J Physiol (Lond) 200:807–819 PubMedCASGoogle Scholar
- Herzberg O, James MNG (1985) Structure of the calcium regulatory muscle protein troponin C at 2.8Å resolution. Nature 313:653–659 PubMedCASGoogle Scholar
- Hill AV (1950) The development of the active state of muscle fibres during the latent period. Proc R Soc Lond [Biol] 137:320–329 CASGoogle Scholar
- Hill TL (1989) Free energy transduction and biochemical cycle kinetics. Springer, Berlin Heidelberg New York Google Scholar
- Hofmann PA, Metzger JM, Grieser ML, Moss RL (1990) Effects of partial extraction of light chain 2 on the Ca 2+ -sensitivities of isometric tension, stiffness, and velocity of shortening in skinned skeletal muscle fibres. J Gen Physiol 95:477–498 PubMedCASGoogle Scholar
- Holmes KC, Popp D, Gebhard W, Kabsch W (1990) Atomic model of the actin filament. Nature 347:44–49 PubMedCASGoogle Scholar
- Homsher E, Millar NC (1990) Caged compounds and striated muscle contraction. Ann Rev Physiol 52:875–896 CASGoogle Scholar
- Huxley AF (1957) Muscle structure and theories of contraction. Prog Biophys Chem 7:255–318 CASGoogle Scholar
- Huxley AF (1980) Reflections on muscle. The Sherrington lectures XIV. University Press, Liverpool Google Scholar
- Huxley AF, Niedergerke R (1954) Interference microscopy of living muscle fibres. Nature 173:971–973 PubMedCASGoogle Scholar
- Huxley AF, Simmons RM (1973) Mechanical transients and the origin of muscular force. Cold Spring Harbor Symp Quant Biol 37:669–680 CASGoogle Scholar
- Huxley AF, Taylor RE (1958) Local activation of striated muscle fibres. J Physiol (Lond) 144:426–441 PubMedCASGoogle Scholar
- Huxley HE (1957) The double array of filaments in cross-striated muscle. J Biophys Biochem Cytol 3:631–648 PubMedCASGoogle Scholar
- Huxley HE (1963) Electron microscope studies on the structure of natural and synthetic protein filaments from striated muscle. J Mol Biol 7:281–308 PubMedCASGoogle Scholar
- Huxley HE (1969) The mechanism of muscular contraction. Science 164:1356–1366 PubMedCASGoogle Scholar
- Huxley HE (1973) Structural changes in the actin and myosin containing filaments during contraction. Cold Spring Harbor Symp Quant Biol 37:361–376 CASGoogle Scholar
- Huxley HE (1979) Time resolved x-ray diffraction studies on muscle. In: Sugi H, Pollack GH (eds) Cross-bridge mechanism in muscle contraction. University Park Press, Baltimore, pp 391–405 Google Scholar
- Huxley HE, Faruqi AR (1983) Time-resolved x-ray diffraction studies on vertebrate striated muscle. Annu Rev Biophys Bioeng 12:381–417 PubMedCASGoogle Scholar
- Huxley HE, Faruqi AR, Kress K, Bordas J, Koch MHJ (1982) Time-resolved x-ray diffraction studies of the myosin layer-line refilections during muscle contraction. J Mol Biol 158:637–684 PubMedCASGoogle Scholar
- Huxley HE, Hanson J (1954) Changes in the cross-striations of muscle during contraction and stretch and their structural interpretation. Nature 173:973–976 PubMedCASGoogle Scholar
- Huxley HE, Simmons RM, Faruqi AR, Kress M, Bordas J, Koch MHJ (1983) Changes in the x-ray reflections from contracting muscle during rapid mechanical transients and their structural implications. J Mol Biol 169:469–506 PubMedCASGoogle Scholar
- Imagawa T, Smith JS, Coronado R, Campbell KP (1987) Purified ryanodine receptor from skeletal muscle sarcoplasmic reticulum is the Ca 2+ -permeable pore of the calcium release channel. J Biol Chem 262:16636–16643 PubMedCASGoogle Scholar
- Irving M, Lombardi V, Piazzesi G, Ferenczi MA (1992) Myosin head movements are synchronous with the elementary force-generating process in muscle. Nature 357:156–158 PubMedCASGoogle Scholar
- Ishijima A, Doi T, Sakurada K, Yanagida T (1991) Subpiconewton force fluctuations of actomyosin in vitro. Nature 352:301–306 PubMedCASGoogle Scholar
- Jewell BR, Wilkie DR (1960) The mechanical properties of relaxing muscle. J Physiol (Lond) 152:30–47 PubMedCASGoogle Scholar
- Kabsch W, Mannherz HG, Suck D, Pai EF, Holmes KC (1990) Atomic structure of the actin: DNAse I complex. Nature 347:37–44 PubMedCASGoogle Scholar
- Katz AM (1979) Role of the contractile proteins and sarcoplasmic reticulum in the response of the heart to catecholamines: a historical review. Adv Cyclic Nucleotide Res II:303–343 Google Scholar
- Kim HW, Steenaart NAE, Ferguson DG, Kranias EG (1990) Functional reconstitution of the cardiac sarcoplasmic reticulum Ca 2+ -ATPase with phospholamban in phospholipid vesicles. J Biol Chem 265:1702–1709 PubMedGoogle Scholar
- Kress M, Huxley HE, Faruqi AR, Hendrix J (1986) Structural changes during activation of frog muscle studied by time-resolved x-ray diffraction. J Mol Biol 188:325–342 PubMedGoogle Scholar
- Lehmann W, Craig R, Vibert PJ (1994) Ca 2+ -induced tropomyosin movement in Limulus thin filaments revealed by three-dimensional reconstruction. Nature 368:65–67 PubMedGoogle Scholar
- Lomo T, Westgaard RH, Dahl HA (1974) Contractile properties of muscle: control by pattern of muscle activity in the rat. Proc R Soc (Lond) B 187:99–103 Google Scholar
- Lowey S, Waller GS, Trybus KM (1993) Skeletal muscle myosin light chains are essential for physiological speeds of shortening. Nature 365:454–456 PubMedGoogle Scholar
- MacLennan DH, Brandl CJ, Korczak B, Green NM (1985) Amino-acid sequence of a Ca 2+ +Mg 2+ -dependent ATPase from rabbit muscle sarcoplasmic reticulum, deduced from its complementary DNA sequence. Nature 316:696–700 PubMedCASGoogle Scholar
- MacLennan DH, Wong PTS (1971) Isolation of a calcium-sequestring protein from sarcoplasmic reticulum. Proc Natl Acad Sci U S A 68:1231–1235 PubMedGoogle Scholar
- Maéda Y, Matsubara I, Yagi N (1979) Structural changes in thin filaments of crab striated muscle. J Mol Biol 127:191–201 PubMedGoogle Scholar
- Matsubara I, Yagi N (1978) A time-resolved X-ray diffraction study of muscle during twitch. J Physiol (Lond) 278: 297–307 PubMedCASGoogle Scholar
- McKillop DFA, Geeves MA (1993) Regulation of the interaction between actin and myosin subfragment 1: evidence for three states of the thin filament. Biophys J 65:693–701 PubMedCASGoogle Scholar
- Melzer W, Herrmann-Frank A, Lüttgau HCh (1995) The role of Ca 2+ ions in excitation-contraction coupling of skeletal muscle fibres. Biochim Biophys Acta 1241:59–116 PubMedGoogle Scholar
- Melzer W, Schneider MF, Simon BJ, Szucs G (1986) Intramembrane charge movement and calcium release in frog skeletal muscle. J Physiol (Lond) 373:481–511 PubMedCASGoogle Scholar
- Milligan RA, Flicker PF (1987) Structural relationships of actin, myosin, and tropomyosin revealed by cryo-electron microscopy. J Cell Biol 105:29–39 PubMedCASGoogle Scholar
- Parry DAD, Squire JM (1973) The role of tropomyosin in muscle regulation: analysis of the x-ray diffraction patterns from relaxed and contracting muscles. J Mol Biol 75:33–55 PubMedCASGoogle Scholar
- Peachey LD (1965) The sarcoplasmic reticulum and transverse tubules of the frog’s sartorius. J Cell Biol 25 (part II):209–231 PubMedGoogle Scholar
- Persechini A, Stull JT, Cooke R (1985) The effect of myosin phosphorylation on the contractile properties of skinned rabbit skeletal muscle fibres. J Biol Chem 260:7951–7954 PubMedCASGoogle Scholar
- Pette D, Vrbová G (1992) Adaptation of mammalian skeletal muscle fibres to chronic electrical stimulation. Rev Physiol Biochem Pharmacol 120:116–202 Google Scholar
- Philipps GN Jr, Fillers JP, Cohen C (1986) Tropomyosin crystal structure and muscle regulation. J Mol Biol 192:111–131 Google Scholar
- Portzehl H, Caldwell PC, Rüegg JC (1964) The dependence of contraction and relaxation of muscle fibres from the crab Maia squinado on the internal concentration of free calcium ions. Biochim Biophys Acta 79:581–591 PubMedCASGoogle Scholar
- Prosser CL (1960) Comparative physiology of activation of muscles with particular attention to smooth muscles. In: Bourne GH (ed) The structure and function of muscle. Structure, part I, vol 2. Academic, New York, pp 387–434 Google Scholar
- Rayment I, Holden HM, Whittaker M, Yohn CB, Lorenz M, Holmes KC, Milligan RA (1993b) Structure of the actin-myosin complex and its implications for muscle contraction. Science 261:58–65 PubMedCASGoogle Scholar
- Rayment I, Rypniewski WR, Schmidt-Base K, Smith R, Tomchick DR, Benning MM, Winkelmann DA, Wesenberg G, Holden HM (1993a) Three-dimensional structure of myosin subfragment-1: a molecular motor. Science 261:50–58 PubMedCASGoogle Scholar
- Reedy MK (1993) Myosin-actin motors. The partnership goes atomic. Structure 1:1–15 PubMedCASGoogle Scholar
- Reedy MK, Holmes KC, Tregear RT (1965) Induced changes in orientation of the cross-bridges of glycerinated insect flight muscle. Nature 207:1276–1280 PubMedCASGoogle Scholar
- Rios E, Brum G (1987) Involvement of dihydropyridine receptors in excitation-contraction coupling in skeletal muscle. Nature 325:717–720 PubMedCASGoogle Scholar
- Rüegg JC (1992) Calcium in muscle contraction. 2nd edn. Springer, Berlin Heidelberg New York Google Scholar
- Rüegg JC, Schädler M, Steiger GJ, Müller G (1971) Effects of inorganic phosphate on the contractile mechanism. Pflugers Arch 325:359–364 PubMedGoogle Scholar
- Sakmann B, Neher E (1983) Single-channel recording. Plenum, New York Google Scholar
- Schneider MF, Chandler WK (1973) Voltage dependent charge movement in skeletal muscle: a possible step in excitation-contraction coupling. Nature 242:244–246 PubMedCASGoogle Scholar
- Schneider MF, Simon BJ (1988) Inactivation of calcium release from the sarcoplasmic reticulum in frog skeletal muscle. J Physiol (Lond) 405:727–745 PubMedCASGoogle Scholar
- Schröder RR, Manstein DJ, Jahn W, Holden H, Rayment I, Holmes KC, Spudich JA (1993) Three-dimensional atomic model of F-actin decorated with Dictyostelium myosin S1. Nature 364:171–174 PubMedGoogle Scholar
- Siemankowski RF, Wiseman MO, White HD (1985) ADP dissociation from actomyosin subfragment 1 is sufficiently slow to limit the unloaded shortening velocity in vertebrate muscle. Proc Natl Acad Sci U S A 82:658–662 PubMedCASGoogle Scholar
- Sleep JA, Hutton RL (1980) Exchange between inorganic phosphate and adenosine 5∩-triphosphate in the medium by actomyosin subfragment 1. Biochemistry 19:1276–1283 PubMedCASGoogle Scholar
- Smith JS, Imagawa T, Ma J, Fill M, Campbell KP, Coronado R (1988) Purified ryanodine receptor from rabbit skeletal muscle is the calcium-release channel of sarcoplasmic reticulum. J Gen Physiol 92:1–26 PubMedCASGoogle Scholar
- Squire JM (1994) The actomyosin interaction-shedding light on structural events: “plus ça change, plus c’est la même chose”. J Muscle Res Cell Motil 15:227–231 PubMedCASGoogle Scholar
- Stull JT, Blumenthal DK, Cooke R (1980) Regulation of contraction by myosin phosphorylation. A comparison between smooth and skeletal muscles. Biochem Pharmacol 29:2537–2543 PubMedCASGoogle Scholar
- Sugi H (1992) Molecular mechanism of actin-myosin interaction in muscle contraction. In: Sugi H (ed) (1992) Muscle contraction and cell motility. Molecular and cellular aspects Springer, Berlin Heidelberg New York, (Advances in comparative and environmental physiology, vol 12) Google Scholar
- Sweeney HL, Stull JT (1990) Alteration of cross-bridge kinetics by myosin light chain phosphorylation in rabbit skeletal muscle: implications for regulation of actin-myosin interaction. Proc Natl Acad Sci U S A 87:414–418 PubMedCASGoogle Scholar
- Takeshima H, Nishimura S, Matsumoto T, Ishida H, Kangawa K, Minamino N, Matsuo H, Ueda M, Hanaoka M, Hirose T, Numa S (1989) Primary structure and expression form complementary DNA of skeletal muscle ryanodine receptor. Nature 339:439–445 PubMedCASGoogle Scholar
- Tanabe T, Beam KG, Powell JA, Numa S (1988) Restoration of excitation-contraction coupling and slow calcium current in dysgenic muscle by dihydropyridine receptor complementary DNA. Nature 336:134–139 PubMedCASGoogle Scholar
- Taylor EW (1979) Mechanism of actomyosin ATPase and the problem of muscle contraction. Crit Rev Biochem Mol Biol 6:103–164 Google Scholar
- Tobacman LS, Sawyer D (1990) Calcium binds cooperatively to the regulatory sites of the cardiac thin filament. J Biol Chem 265:931–939 PubMedCASGoogle Scholar
- Toyoshima YY, Kron SJ, McNally EM, Niebling KR, Toyoshima C, Spudich JA (1987) Myosin subfragment 1 is sufficient to move actin filaments in vitro. Nature 328:536–539 PubMedCASGoogle Scholar
- Trinick J (1991) Elastic filaments and giant proteins in muscle. Curr Opin Cell Biol 3:112–119 PubMedCASGoogle Scholar
- Ulbrich M, Rüegg JC (1971) Stretch induced formation of ATP- [32] P in glycerinated fibres of insect flight muscle. Experientia 27:45–46 PubMedCASGoogle Scholar
- Ulbrich M, Rüegg JC (1976) Is the chemomechanical energy transformation reversible? Pflugers Arch 363:219–222 PubMedCASGoogle Scholar
- Uyeda TQP, Kron SJ, Spudich JA (1990) Myosin step size-estimation from slow sliding movement of actin over low densities of heavy meromyosin. J Mol Biol 214:699–710 PubMedCASGoogle Scholar
- Uyeda TQP, Ruppel KM, Spudich JA (1994) Enzymatic activities correlate with chimaeric substitutions at the actin-binding face of myosin. Nature 368:567–569 PubMedCASGoogle Scholar
- Uyeda TQP, Warrick HM, Kron SJ, Spudich JA (1991) Quantized velocities at low myosin densities in an in vitro motility assay. Nature 352:307–311 PubMedCASGoogle Scholar
- Wagenknecht T, Grassucci R, Frank J, Saito A, Inui M, Fleischer S (1989) Three-dimensional architecture of the calcium channel/foot structure of sarcoplasmic reticulum. Nature 338:167–170 PubMedCASGoogle Scholar
- Wakabayashi K, Tokunaga M, Kohno I, Sugimoto Y, Hamanaka T, Takezawa Y, Wakabayashi T, Amemiya Y (1992) Small-angle synchrotron x-ray scattering reveals distinct shape changes of the myosin head during hydrolysis of ATP. Science 258:443–447 PubMedCASGoogle Scholar
- Webb MR, Hibberd MG, Goldman YE, Trentham DR (1986) Oxygen exchange between Pi in the medium and water during ATP hydrolysis mediated by skinned fibres from rabbit skeletal muscle. J Biol Chem 261:15557–15564 PubMedCASGoogle Scholar
- Weber HH, Portzehl H (1954) The transference of the muscle energy in the contraction cycle. Prog Biophys Mol Biol 4:60–111 Google Scholar
- Xie X, Harrison DH, Schlichting I, Sweet RM, Kalabokis VN, Szent-Györgyi AG, Cohen C (1994) Structure of the regulatory domain of scallop myosin at 2.8 Å resolution. Nature 368:306–312 PubMedCASGoogle Scholar
- Yanagida T, Yoshie H, Ishijima A (1993) Nano-manipulation of actomyosin molecular motors in vitro: a new working principle. TIBS 11:319–324 Google Scholar
- Zorzato F, Fujii J, Otsu K, Phillips M, Green NM, Lai FA, Meissner G, MacLennan DH (1990) Molecular cloning of cDNA encoding human and rabbit forms of the Ca 2+ release channel (ryanodine receptor) of skeletal muscle sarcoplasmic reticulum. J Biol Chem 265:2244–2256 PubMedCASGoogle Scholar
- J. C. Rüegg